Solar Myths and Solar Facts
Solar power is generating a lot of heat, but what the debate needs is a cool-headed look at where solar systems will pay off and where they won't.
On "Sun Day," May 3,1978, President Carter promised renewed vigor in governmental support of solar radiation as a "clean, renewable, and economically competitive energy source." That his speech was made in a pouring rain storm at Golden, Colorado, was perhaps prophetic, for it was anything but a solution to the energy crisis.
"Among all the possibilities, whether simple or complex, low technology or high, stands the sun," begins a pamphlet published by the California Energy Commission. It goes on with encouraging words: "Solar energy is abundant, nonpolluting, and free. It is not affected by changes in international politics or trade, or inflation at home. It is available where it is needed. It is dependable, even in winter. And for at least the next four billion years, the sun will be warming the earth and providing the energy to those who take it."
Such glowing praise for solar power is common in the literature of antinuclear advocates and the environmental movement, as well as among some scientists. But to hope that solar power is the answer to all of our energy needs, that we need worry no more about depletion of fossil fuels or the risks of nuclear power, is to give way to utopianism. "Turn off the nukes, turn on the sun," says the bumper sticker, but one-liners like that will not carry us into the 21st century.
SUNNY STATISTICS
The United States has an area of some 3,615,122 square miles. With solar insolation—meaning the energy that falls on a given area over some period of time—ranging from 100 to 750 gram-calories per square centimeter depending on the time of year and atmospheric conditions, it works out to 171 quads (quadrillion, or 1015, BTUs) of solar energy falling each day. And since our present annual consumption is about 80 quads per year (only 0.22 quads per day), shouldn't we be able to meet all of our energy needs by capturing the sun's energy?
The trouble is that the amount of solar insolation is not really what counts. Those impressive figures in fact translate into a power density of about one kilowatt per square meter at best, during the period when the sun is shining. One kilowatt is enough to run ten 100-watt light bulbs or one iron or toaster.
So why do so many nontechnical people tend to go along with the promotional razzle-dazzle surrounding solar power and consent to having their hard-earned dollars being thrown at that solution by government? Probably because they keep hearing the misleading figures—that the sun is beaming down on us something like 780 times the energy we actually use, for example.
The theme was advanced by Paul Rappaport, then director of the federal government's Solar Energy Research Institute (SERI) in Golden, Colorado, in writing his "Toward a Sun-Powered World" for the 1979 Yearbook of the Encyclopedia Britannica. Downplaying coal and oil, Rappaport brought up the controversial "greenhouse theory," according to which the burning of fossil fuels and the production of carbon dioxide are raising the overall temperature of the earth's atmosphere and "could alter climate and perhaps even precipitate such catastrophic effects of atmospheric heating as the melting of the polar caps and consequent flooding of the world's coastal cities."
To make his advocacy of solar power more pressing, Rappaport continued: "Presently nuclear power seems an unwise bet as a major energy source for the future. Unanswered questions remain regarding reactor safety and nuclear waste disposal." He failed to note that answers have been provided but have been drowned out by antinuclear, soft-technology advocates and the politicians and press who pay attention only to them.
Should we not dismiss Mr. Rappaport's words as an attempt to perpetuate and solidify his bureaucratic position at the time with SERI? Many pronuclear arguments are thus waved away as biased because of their proponents' occupation (it is not fashionable to be employed in the industry and therefore to know what one is talking about). But the argument should not rest on such things. We cannot afford to go with anything less than careful analysis of the facts of the matter, technical and economic.
WHAT THE SUN CAN DO
Mr. Rappaport was not entirely in accord with his own staff in his glowing predictions for solar power. According to SERI's deputy director, even if solar energy could provide from 7 to 10 percent of US energy by the year 2000, it would probably not be able to displace a single existing nuclear or coal-fired power plant. He points out that, while solar may keep our standard of living better than if it did not exist, nuclear and coal would still have to provide most of the nation's electricity supply. About 28 percent of US energy consumption goes into the production of electricity; other principal energy uses are transportation (27 percent), space heating (18.5 percent), and industrial heat and steam (23 percent)—see Figure 1.
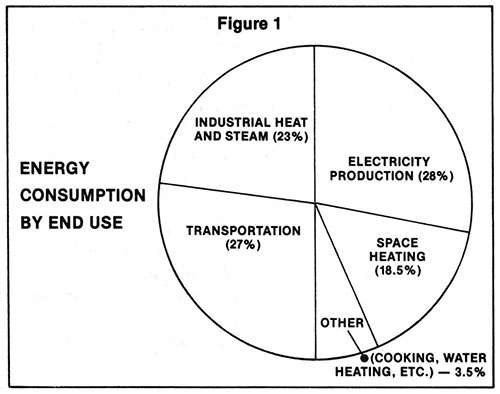
The solarists are on solid ground when they point to water and space heating. Solar energy has been used for centuries for exactly these purposes. And because the nontechnical public can think in those simple terms, they are led to the mistaken conclusion that that's all there is to the energy problem.
Presently, at a cost of several thousand dollars per home, one can install solar collectors in sunny climates that will do a creditable job of heating water and space. The California Building Industry Association (CBIA)—with more than a few dollars of help from the California Energy Commission—has published a hefty looseleaf syllabus distributed at each of its several "Solar Seminars" conducted around California for builders, engineers, and architects.
Robert Kuntz, technical services director for the CBIA and executive secretary of the California Society of Professional Engineers, has built up some of the inflated hopes for solar power in his remarks before these seminars. Alluding to the high capital cost of nuclear plants and the long lead times, and erroneously implying that residential energy consumption is of primary importance (see Figure 2), he creates the impression that solar energy is an alternative to nuclear. In response to a letter challenging him on these points, Kuntz blandly denied the implications. "I am sorry that you misunderstood my remarks concerning nuclear power in the State of California," he wrote. "The California Building Industry Association is in no position to pass judgment on the merits or availability of nuclear power." Yet he proceeded to do just that at the very next seminar.
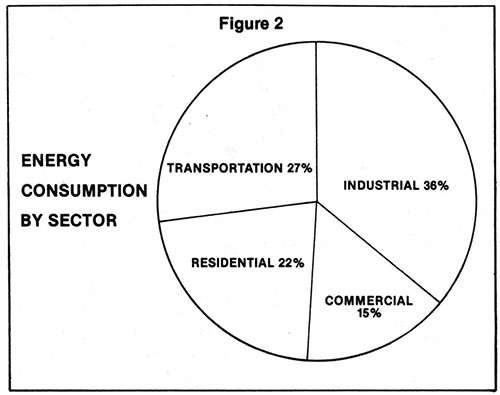
The syllabus, however, does furnish some good information on both passive and active solar heating designs, including several heat-pump systems that have merit. (Active systems are those requiring fans, pumps, and other mechanical equipment to produce and move heat. Passive systems rely on building structure and nonmechanical means—circulation, convection, and radiation—to collect and move heat.)
The sun is for some applications a practical source of energy. But free? By touting solar energy as free, the California Energy Commission does a disservice to the public. Just as the cost of construction, maintenance, and decommissioning is factored into energy cost in a power plant, so is the cost of installation, maintenance, and eventual replacement of a solar system a necessary expense. It might be helpful to illustrate some of the trade-offs involved in, say, a solar water-heating system.
HEATING UP THE H20
Solar heating is actually most adaptable to water, particularly on a retrofit (replacement) basis. Solar space heating is best included when a structure is first built, because many factors, like shape of the roof and overhangs, north-south orientation of the house, insulation, and shading for summer use, have to be taken care of.
While actual hot-water needs depend on personal living habits—the usage of washing machines, dishwashers, etc.—a minimal standard is 20 gallons of hot water, 130 degrees Fahrenheit, per person per day. For a 55-degree inlet temperature this means that, for a family of four, 80 gallons of water weighing 667 pounds must be raised in temperature by 75 degrees, which requires 50,022 BTUs of energy. Add to this the heat losses from the required conventional back-up water heater, typically 26,000 BTUs per day (American Gas Association estimate), and one obtains 76,022 BTUs per day as the heat requirement for hot water for a typical family of four.
Solar water heating systems costing $2000-$4,000 (installed) can deliver to the water system (after all heat losses are taken into account) about 50,000 BTUs on a good day in, say, the Los Angeles area. So something like two-thirds of the water-heating energy requirements can be furnished by readily available solar water-heating systems. The resultant saving in BTUs amounts to about 17 kilowatt hours (KWH) if one assumes 90 percent efficiency for an electric hot-water system. The saving in electricity cost is something like 85 cents per day. From this must be subtracted the cost of electricity for pumps to operate the solar system if it is not entirely passive (which it usually isn't). This is typically about 3 cents per day, so the net saving comes out to about 82 cents per day in fuel cost assuming electricity as the alternative.
For natural gas or propane as the alternative, the saving is somewhat less. For propane at 44 cents per gallon and with a higher heating value of 91,000 BTUs per gallon, 50,000 BTUs at 40 percent efficiency costs 60 cents versus 85 cents for electricity. Natural gas costs much less, but since this is only because of artificial price controls, this is not a fair comparison (at least if we're interested, as many solar advocates are, in energy conservation on a national scale).
LONG-TERM COSTS
But now we must add in the costs of maintaining and ultimately replacing the system. According to Sunset Books' Homeowner's Guide to Solar Heating, a study in the San Diego area found a lifecycle cost of $5,050 for electric water heating versus $2,689 for a solar system. These figures, however, do not include some important factors, and the analysis is based on a 20-year life for the (assumed) $1,500 solar system. There is no reason to believe that the problems with rust, hard-water deposit build-up, pump bearings and impellers, contactor contacts, etc., will be any less with the solar system. A 10-year life would be more realistic and certainly more conservative, which brings the life-cycle cost down to $2,525 for electric and $2,132 for solar, other assumptions of the study being the same.
More realistically, one must include the cost of capital—what one could earn on the investment during the life of the equipment if that money were turned over in, say, gold, diamonds, treasury bills, or real estate. And a $3,500 installed price for the solar system is more realistic, especially if one seeks a full 75,000 BTUs per day. If approximately equal maintenance costs are assumed, a comparison between solar and propane comes out about as follows:
Propane
First cost: $350
10-year capital cost*: 737
10-year energy cost**: 3,490
10-year life-cycle cost: $4,577
Solar
First cost: $3,500
10-year capital cost*: 7,371
10-year energy cost**: 175
10-year life-cycle cost: $11,046
* Total earnings at 12 percent compounded annually for 10 years.
** Based on energy price increases of 10 percent per year, starting with 60 cents per day for propane and 3 cents per day for solar, for the electricity for pumps, etc. Maintenance costs assumed equal.
Had the alternative been electric, the energy cost would have risen by $1,454 and the comparison becomes $5,565 for electric versus $11,046 for solar. These comparisons do ignore the availability of solar tax credits, which vary from state to state and can markedly affect the outcome. In California, one of the most liberal states, there is a 55 percent credit against personal income taxes for capital expenditures on solar equipment up to $3,000. In the present example, that would change the 10-year cost of the $3,500 solar water heating system as follows:
Net first cost: $1,850
10-year cost of capital: 2,949
10-year energy cost: 175
$4,974
Thus, with the tax credit the solar system is less costly than the electric one, and only slightly more costly than the propane one.
So whether there is an economic advantage to a solar hot-water system depends on the life cycle, assumptions about price increases and the cost of capital, the possible existence of tax credits, and above all on how many hours the sun shines each year. If one is concerned about the availability of an alternate energy source, the often higher cost of solar might be justified. But even if everyone converted, the national energy problems wouldn't be solved that easily!
MULTIPLE NEEDS
A 1976 study by the Energy Research Group in Framingham, Massachusetts, provides some revealing data. Even if the solar industry could be expanded to provide heating for 100,000 buildings a year in 1990 and 600,000 to 2 million buildings a year by 2000, only one to three percent of total US energy needs could be met by the year 2000. If all 72 million households in the United States could be converted to solar water heating overnight, it would save three percent—about 2.5 quads—of our national energy requirements. While this is nothing to sneeze at—the 1973 oil embargo created a one-quad energy shortfall and contributed to the greatest economic recession in 40 years—we are facing a need for anywhere from 20 to 90 more quads per year by the year 2000 as the population increases (experts vary widely in their projections and assumptions). Whatever the exact figure, this additional energy need is enormous and cannot be met by solar systems trying to extract energy at the dilute power density of one kilowatt per square meter.
The real problems of energy are not water and space heating, indeed, not even transportation, in spite of gas lines that can be traced directly to government mismanagement. We must not allow ourselves to be misled by solarist arguments based on water-and space-heating uses alone. As we have seen, 27 percent of our energy use is in transportation and another 28 percent goes to produce electric power.
We already use solar power extensively in making electricity. Hydroelectric power is, after all, solar in origin, since it depends on rainfall; snowfall; and evaporation, condensation, and pressure cycles, the energy for which comes from the sun.
We may be able to find a supply of fuels that can economically supplement fossil fuels to some extent, but this is likely to be tiny compared to our needs. Certainly, we can produce methane from garbage, steam from fumaroles, fuel from peat, alcohol from wood and corn, and so on, but these activities are productive only because energy from the sun has been integrated and stored in the basic substances over some past period of time. But if we talk about direct solar energy use and hope to be able to use solar systems to replace even our nuclear capacity—now on the order of some seven quads per year in terms of energy consumption (taking into account plant efficiency)—we are setting ourselves up for a tragic collision with reality.
And yet this is precisely where President Carter has us headed, judging by his pronouncements on energy in July 1979 after his guru sessions at Camp David. The "July Massacre" of his cabinet saw a technically knowledgeable James Schlesinger replaced by a former Coca Cola executive as energy secretary, but no matter—policy actually remains unchanged from prior failures: throw money at it, and the problem is solved.
The president wants solar power to furnish 20 percent of US energy needs by the year 2000, he says, and all he needs to do this is the National Solar Bank. Quite possibly Dr. Schlesinger's demise resulted from his adherence to the technical facts, which show this to be an impossible goal unless the overall consumption of energy is cut so drastically as to result in the nation's coming to a standstill. One can, after all, convert 5 percent into 20 percent by quartering the base upon which the percentage is calculated.
In any event, Mr. Carter will assuredly not be president in the year 2000 if we make it that far, so nobody will remember whether he achieved his goal or not. One thing is certain: the production of energy is not as simple as the production of Coca Cola, and reshuffling the energy czars in Washington is not the solution.
PLUGGING IN TO SOLAR
Electricity is the major problem. With cheap, abundant electric power, even transportation fuels could be produced economically by extracting and liquefying hydrogen from sea water—although this would require a "plutonium-hydrogen economy," the first part of which raises images of disaster for opponents of nuclear power.
A recent issue of Electronics (July 19, 1979) ran a special feature on the state of the art in photovoltaics, whereby solar cells use the sun's light rather than heat to transform solar energy into electricity. SERI's former director would have us spend $1.5 billion on such devices over a 10-year period. Although some experimental (and expensive) laboratory designs have reported efficiencies of 16-20 percent, the average for mass-produced solar cells still stands at around 8-10 percent. The cost, while it has been dropping, is still (1980) in the vicinity of $10,000 per peak kilowatt for the cells alone.
The highest reported output of a solar cell is the open-circuit 0.655 volt reported for an Australian experimental design. So there are formidable technological problems to be overcome before solar cells could produce the typically 300,000 volts of three-phase alternating current used for long-distance transmission lines to reach urban areas. It is even far beyond the present state of the art simply to obtain the 240 volts AC that most 150-ampere households have now in three-wire systems.
But the problem does not stop there. Energy engineer Dr. Petr Beckmann estimates that a 1,000-megawatt solar electric plant—whether photovoltaic or thermal—would require some 50 square miles of land area—32,000 acres—to generate the electricity that can be produced by a nuclear or fossil plant on 25 acres. (A 1,000-MW plant can normally serve a city of one million people.) Even this does not allow for the increased land area required for longer transmission lines between the sunny deserts most suitable for solar plants and the population centers using the power. Nor does it allow for the necessary access roads, rights of way, and substations or raise the issue of the cost of building those longer lines.
And there is still another element in the equation. According to SERI's Dr. Kathryn A. Lawrence, a 1,000-MW solar electric plant would require 35,000 tons of aluminum, 2 million tons of concrete, 7,500 tons of copper, 600,000 tons of steel, 75,000 tons of glass, 1,500 tons of chromium and titanium, and 5 tons of silver, and more. Such a plant would require close to 20,000 hours of operation to return the energy required to build it. The tonnage of structural metals alone is 1,056 times as great as for a nuclear or fossil plant of the same size.
Suppose, however, that we were to attempt to try to meet all of our year-2000 electricity needs of up to 43 quads per year with solar power plants. What would that require in land area? Using 1,000-MW solar plants operating 10 hours per day (a generous assumption for the sunshine period) at 90 percent availability (only 36.5 days per year for maintenance, such as cleaning the snow, dirt, and bird droppings off the collectors), translates into 0.0112 quad per year per plant. To produce 43 quads this way would require 3,825 plants, or a minimum of 191,250 square miles of real estate. This is an area about 58 percent more than the entire area of New Mexico, 68 percent more than the entire state of Arizona, and even 72 percent of the total area of Texas!
Can any of the environmental experts estimate the ecological damage that would result from placing this much of the United States into permanent shadow? Even the august Sierra Club has some doubts about this—in its Towards an Energy Policy it cautiously admits that "there may be serious environmental problems with large [solar] systems. They may be wasteful of open land and material resources."
DO-IT-YOURSELF POWER
It seems rather obvious that centralized solar electric power is enormously impracticable, a fact apparently lost on the DOE, which has just put on line a $100,000-per-kilowatt installation in Arizona and plans to spend $105.5 million on a 10-MW facility in Barstow, California. But what about smaller, individual systems? Might we not get rid of that old utility bill this way and contribute to solving the energy shortage?
Solar-cell panels presently available (for example, the Spectrolab 12-volt, 96-watt array delivering 6 amperes, 26.8 ampere-hours per day under average sun conditions) have a usable output of about 5.58 watts per square foot of area. So let's examine the possibility of putting an array of these panels on the house roof and perhaps spilling over on to the barbecue area and the back yard. Let's assume that we don't have that space already taken up by solar panels for heating water or space (there isn't room for both). What sort of installation would we need, and how much would it cost?
Present technology will let us assemble possibly 100 such panels, to which one would have to devote 1,722 square feet of south-facing roof and yard. Hooked up in series-parallel, 100 panels of this type can deliver 120 volts direct-current at 60 amperes, 32 KWH per average day, which electricity would cost $1.61 when purchased from a utility at 5 cents per KWH.
The problem is, the present cost of such an array would be $48,719 plus sales tax (although some states exempt solar equipment from sales tax)—about $53,000 with installation and a high-powered solid-state inverter to convert it to square-wave 60-cycle AC. Now motors and TV sets don't operate too well on square waves, but they will run that way. We could probably add another $500 or so of filter to make them look more like sine waves, but then we would lose some in energy efficiency. So we can amortize our investment in a little over 90 years, if the house roof holds up that long!
Of course, the cost of solar panels will come down as technological developments occur, but what does it have to come down to? Assuming the output per unit area would double as a result of such progress, we could save $3.20 per day in electricity cost. This could possibly justify an investment of $6,784 (again using a 12 percent cost of capital)—which means that the solar panels themselves would have to drop to about $2,500 or about $347 per peak kilowatt before installation, as against the present price of $6,766 per kilowatt. The DOE's most optimistic target is $2,800 per kilowatt with the start of automated mass production in 1982, down to $500 per kilowatt by 1990 (as reported in Electronics in July 1979).
But this isn't the whole story by any means. It neglects the cost of maintenance and assumes a trouble-free system. A few substantial short-circuits, and the most expensive part of an inverter that isn't well enough protected will vanish in a cloud of smoke. Let lightning strike the collector panels, and the whole array might have to be replaced—if one is to insure against this risk, then the cost of that insurance must be figured into the equation. And, of course, the little devils across the street with their new birthday .22s will have a great time using the solar panels for target practice. Or their new slingshots or baseballs will do as well.
And finally, for the hours that the sun doesn't shine, one must rely on the utility after all. For the lifestyle that can afford even the $6,784 investment for the system, household electrical needs come out to more like 45 KWH per day than the 32 KWH delivered by the system. Piet B. Bos, director of the Solar Energy Program of the Electric Power Research Institute, talks about this capacity displacement problem. "Capacity displacement is the joker in the solar deck," he says in his article "Solar Realities." "The solar advocates argue that, once developed, energy from the sun could easily provide a major part of the energy the nation needs and eventually could do it at a price as low as any other fuel system. But what happens if the sun doesn't shine for a week? The answer is that we must then fall back on conventional energy sources until that cheap solar source is again available." Bos points out that the utilities must therefore maintain standby capacity to make up these deficits. That idle capacity is costly, and "all the expenses of the backup plants would have to be added to the price of energy from solar power."
SAFER RADIATION?
But isn't solar safer? Here again, consumers are being conned by optimists who don't know or don't care about the solar facts. R. Du Val of the San Francisco Operations Office of DOE noted a few months ago that solar cells contain arsenic, cadmium, and other carcinogens. "What happens if there is a fire?" he asked, and "How will old solar cells be disposed of?" So the "totally benign" environmental concept of solar energy needs further evaluation and is in fact being studied by DOE, he says.
Dr. Beckmann has pointed out many times in the Access to Energy newsletter that massive shifts to individual solar energy systems could result in many deaths—from people falling off their roofs when cleaning off the collectors, for example. He points out that accidental falls kill about 16,500 Americans a year, added to about 1,300 more killed by falling objects, making this category the number-two accidental killer in the United States (automobile accidents being number one). Homeowners will be climbing on the roofs to clean off the snow and dirt and leaves after every storm, and the risk from this can be estimated, but it is obviously orders of magnitude higher than the deaths per billion megawatt-hours that occur for other energy cycles.
And even this does not consider the accidents with storage facilities, sulfuric acid in storage batteries, or the explosive hydrogen-oxygen mixture from charging them, and so on, all in areas where children may play. Is being free of that "greedy old utility" and that ''nasty old uranium" really worth this risk?
A CLOUDED FUTURE
And would it deliver so much independence, after all? Electricity is what powers our industrial machine. Without it, the motors don't run, the lights don't work, the semiconductors don't grow, the conveyors don't move. Production stops. Without electricity, much of our food isn't grown. Without transportation fuel, nothing gets to market (observe the panic reactions in Washington and state capitols when the independent truckers struck to get more diesel fuel and permission to pass on its higher cost to their customers). Horses? Perhaps, but using 1918 technology just to achieve today's US level of agricultural production—which feeds most of the world—would take, according to a Department of Agriculture official, 61 million horses and mules. And that would take 20 years to breed from the 3 million now alive, and it would use up half the US cropland just to feed them.
Those with high hopes for solar power forget that the production and distribution of food is energy-intensive. A glass of milk requires a half-glass of diesel fuel to reach the table. It takes a half-pound of coal to produce a one-pound loaf of bread, three pounds of coal for a pound of hamburger, and so on. It is through energy that the United States has the highest agricultural output in the world, with one agricultural worker feeding 59 people against the world average of 5.1. (And this, by the way, is why it is such nonsense to bandy about the now-familiar comparison—that the United States has such a small percentage of the world's population but accounts for such a large percentage of its energy consumption.)
The energy needs of our modern society simply cannot be met with sunbeams and windmills alone. They cannot be met, in fact, with all of the energy we can possibly produce in 20 years, because of the history of the politicization of energy. The gas lines in 1974 and 1979 are simply the forerunner of things to come. When we get to rolling blackouts in electricity because of the refusal of politicians and regulators to listen to reason instead of to the stentorian polemics of pseudo-scientists and extremists among those concerned about the environment, we will discover, too late, what a shortfall in energy really means.
Consumers are, at best, hearing half-truths about the prospects for solar energy. And several years down the road?—when a disillusioned public comes to realize that solar is impracticable for mass-produced electric power? and that other options have by that time been foreclosed? What we are likely to see then is a backlash against solar power, not unlike the one unleashed in the past few years against nuclear power, after decades of government and industry PR that minimized the cost and complexity of nuclear-power production. If there is going to be "a sunny side of the street," we must assess our present solar possibilities realistically. And if we do, we see that sunshine cannot be the answer to our energy woes.
R.W. (Bill) Johnson is a professional electrical engineer with a private consulting practice in California. He is the author of several books, numerous articles, and a periodic technology column for a local weekly. He authored "The Energy Crisis and How to Solve It" for the May 1975 issue of REASON.
Solar History at a Glance
Prehistory Ancient man dried crops, baked bricks, distilled liquids, with solar heat.
212 BC: Archimedes used reflected sunlight to burn sails of enemy ships.
1600s: Holland used windmills to pump sea water.
1774: Joseph Priestley used crude solar furnace in discovery of oxygen.
1872: Solar distillery in the northern Chile desert provided fresh water from salt water at nitrate mine.
1878: Solar heated boiler ran steam engine to operate printing press at Paris exhibition.
1902-1911 Solar engines up to 20 horsepower built at St. Louis, Philadelphia, and Needles, California.
1913: Solar engines pumped water and generated electricity in Egypt.
1920: Solar water heaters used in Florida.
1939: Massachusetts Institute of Technology experimented in solar home heating.
1950: Major symposium on solar home heating.
1959: Harry Thomason built prototype of first economical, successful solar-heated home.
1961: Report to United Nations on Mexican solar cooking tests.
1967: Silicon solar cels powered isolated Japanese radio repeater stations.
1968: Solar furnace capable of generating one megawatt of power daily built in France.
1973: Energy crisis stimulated interest in solar energy.
1974: Congress passed Solar Heating and Cooling Demonstration Act.
1975: Solar Energy Industries Association created.
1976: Government launches solar energy programs; plans announced for solar housing development.
This article originally appeared in print under the headline "Solar Myths and Solar Facts."
Hide Comments (0)
Editor's Note: As of February 29, 2024, commenting privileges on reason.com posts are limited to Reason Plus subscribers. Past commenters are grandfathered in for a temporary period. Subscribe here to preserve your ability to comment. Your Reason Plus subscription also gives you an ad-free version of reason.com, along with full access to the digital edition and archives of Reason magazine. We request that comments be civil and on-topic. We do not moderate or assume any responsibility for comments, which are owned by the readers who post them. Comments do not represent the views of reason.com or Reason Foundation. We reserve the right to delete any comment and ban commenters for any reason at any time. Comments may only be edited within 5 minutes of posting. Report abuses.
Please
to post commentsMute this user?
Ban this user?
Un-ban this user?
Nuke this user?
Un-nuke this user?
Flag this comment?
Un-flag this comment?