No More Doomsday
"Limits to growth" is a myth-but there are still plenty of good reasons for going into space.
The Space Age is commonly thought to have begun in October 1957, when the Soviet Union launched the first artificial satellite of the earth as part of the International Geophysical Year. Yet the following decade of space exploration by both the Soviet Union and the United States had little effect on the average person's understanding of the world in which we live—until the flight of Apollo 8 in December 1968. Prior to that mission, no one had ever thought to send a camera deep into space and turn it back to look at the whole planet earth in a single picture. The pictures taken during that mission finally propelled the modern world into the Space Age, for our ways of thinking about the world were drastically and irreversibly changed.
Two long-standing issues in the industrialized world were sharply affected by the wide circulation of the Apollo pictures. One was environmental damage from human activities, especially from industrial and agricultural pollutants. These issues had been addressed by such books as Fairfield Osborn's Our Plundered Planet (1948) and Rachel Carson's Silent Spring (1962). The other issue was the question just how many people the earth can support, especially if we all want to enjoy the material affluence of the industrialized nations. This question had been raised by Thomas Robert Malthus at the end of the 18th century; during the 1950s and '60s it had been discussed mostly by such groups as Planned Parenthood and was the subject of a few books such as William and Paul Paddock's Famine 1975 (1967) and the first edition of Paul R. Ehrlich's The Population Bomb (1968).
But none of this had had any significant impact on the average man or woman in the street in the industrialized countries, until the Apollo 8 pictures brought back vivid and convincing proof that the biosphere of the earth is a single, integrated, and interdependent system in which the use of pesticides in Kansas can indeed affect the fertility of penguins in Antarctica. In the context of the vast scales of the lifeless universe surrounding the earth, the biosphere looked very fragile and delicate. Almost overnight, the ecology/environment movement became a powerful political force. The environmentalists quickly adopted Buckminster Fuller's phrase "Spaceship Earth" as a reminder that the survival of humanity depends on the health of the biosphere just as much as the survival of the astronauts depended on the proper functioning of Apollo's life-support systems.
The 1970s have been marked in the United States by a high degree of environmentalist activism, beginning with the creation of the Environmental Protection Agency and the passage of the Clean Air Act in 1970. The American SST program was killed, in part, because of concern about the effects its exhaust emissions might have on the ozone layer. Environmental Impact Statements became a necessary part of planning major construction projects, often resulting in extensive court battles. The movement to prevent further deployment of nuclear power plants achieved some significant successes. Fluorocarbon propellants have been banned from aerosol products; lead additives have been banned from use in new cars; and DDT is no longer generally available. The issue of environmental damage by human activities obviously became popularized and politicized.
While the other issue has never reached such a wide audience, it has continued to be debated in the halls of academe, in some governmental offices, and in dedicated circles among the environmentalists. In part, the narrow interest in the long-term survival of humanity is due precisely to the long time scales involved, ranging from a few decades to centuries or more. The more predominant and pessimistic side of this debate is represented by a stream of thinking that can be referred to as the "Doomsday Mystique." It has become accepted as part of the conventional wisdom of the present age by most people in the circles mentioned above.
The Doomsday Mystique has been most completely embodied in The Limits to Growth (1972), the Club of Rome's report describing the results of a pessimistic computer projection for the global socioeconomic system to the end of the next century. This report, which has since sold several million copies worldwide, projected a catastrophic collapse of industrialized civilization around the world in the next 50 to 80 years due to exhaustion of mineral resources, escalating pollution levels, and massive famine due to overpopulation. Its conclusion was that the only escape is to abandon standard industrialized consumption patterns, recycle everything, and institute stringent worldwide population controls. Similar themes may be found in the current literature of most environmentalist action groups and various organizations promoting zero population growth.
The Doomsday Mystique can be characterized by a set of beliefs about the world that have become so unquestionably accepted by its adherents as to have the strength of axioms. These axioms of the conventional wisdom of our age are:
• World food production is already at its limits. Increased production must necessarily create major ecological dislocations and damage.
• Pollution of the environment increases directly as the number of people increases and much faster as wealth per person increases.
• Reducing famine (by increasing food per person) will accelerate population growth, compounding the global problem.
• Birth rates can be reduced only by universal access to birth control technologies, with thorough education on the necessity and methods of use.
• Nonrenewable resources (especially for energy) will be depleted very soon (in no more than a few decades).
But more careful analyses of these points have emerged in the last few years, suggesting that these axioms are, at best, grievous oversimplifications.
FOOD FOR ALL Perhaps the best currently available analysis of global food production is a book by Frances Moore Lappe and Joseph Collins, Food First! (Houghton-Mifflin, 1977). No country on earth today, they show, is so lacking in necessary resources that it could not feed itself abundantly provided certain social, legal, and political arrangements are changed. With the necessary changes, food production can increase far more rapidly than through expansion of cultivated acreage or through technological changes in agricultural methods. Bangladesh, for example, now has cultivated acreage per person twice that of Taiwan; yet no one is starving in Taiwan, while Bangladesh is considered one of the most impoverished and famine-stricken countries in the world. The same comparison holds for India and China.
How has starvation been banished from China, Taiwan, and South Korea, to name a few countries in which it was endemic 25 years ago? Basically, through changing patterns of land ownership. Farms owned and operated by families or small cooperatives are far more productive, acre for acre, than giant farms using mechanized agriculture, expensive fertilizers and pesticides, and improved seeds. Small farmers in Argentina, Brazil, Chile, Colombia, Ecuador, and Guatemala have been shown to produce from 3 to 14 times as much per acre as do large farmers. The small farmer obtains these results by substituting immense quantities of hard labor for the technological inputs he cannot afford, by planting closer together than machines would, and by interplanting and rotating complementary crops.
This is not to say that the Green Revolution is a bad thing, but it has only been successful where agriculture is primarily carried out on family-owned small farms rather than on very concentrated landholdings. The Green Revolution has been successful in the Punjab State of India, where land ownership is widely distributed among the population. (The wide distribution of agricultural land in the United States during the 1930s and '40s was essential to the success of the new hybrid varieties of corn.)
In other parts of the world, a major factor in famine is large landholders' cash cropping for export. Throughout the African Sahel, during the worst years of the drought in the early 1970s, the total production of peanuts for export actually increased. And it is governmental policies that favor export crops over food crops for domestic consumption. These policies are thus a major problem, which can be remedied quickly if the political will to do so can be mustered.
Technological means of improved food storage and preservation, on the other hand, do offer prospects for major improvements in food supply. Estimates of grain losses to vermin (primarily rats) in Third World countries such as India range from about 25 percent to as high as 70 percent. Reduction of these losses would provide significant gains in effective food production without ecological damage (except to the rat population).
Finally, innovations in technology can provide large improvements in agricultural yields while actually reducing ecological impact. Closed-environment agriculture using hydroponic techniques, additional artificial lighting, and enhanced carbon dioxide concentrations in the air inside can increase yields per acre by factors as large as 400. These methods can be either highly capital-intensive or highly labor-intensive, providing reasonable matches to supplies of capital and labor for both the industrialized and the Third World nations. (Space colonies, of course, will build on these techniques extensively. Agriculture may yet derive important spin-offs from the space program in a nuts-and-bolts sense.)
AFFLUENCE POLLUTES? Despite all the clamor about the rape of the environment in the industrialized nations, the historical fact is that a higher degree of industrialization and technology has tended to reduce adverse environmental impact per person, although the specific types of pollution have changed with time. Of all human pollutants, the potentially most lethal form is fecal contamination of water. This is the primary method of transmission for such nasty diseases as typhus, cholera, dysentery, hepatitis, and polio.
With growing affluence, the industrialized countries have invested large sums of capital to control sewage, and this has been the major factor in improving public health (and especially infant mortality) during the last century. In poor countries today, these diseases are still endemic. (Polio and hepatitis are peculiar cases. These diseases, if contracted in infancy, are usually quite benign. In countries with poor sanitation, virtually everyone is exposed in infancy and acquires lifelong immunity. In countries with good sanitation, most of the adult population is vulnerable to these diseases, which are much more virulent in adults. Even before polio vaccines were developed, however, the worst polio epidemics were far less damaging to industrialized nations than are average outbreaks of cholera and dysentery in the Third World today.)
Air pollution from our industries and automobiles does pose problems at the present time, but these have been generally overrated. Contrary to widespread belief, it cannot be shown that even the great "London killer fogs" of the 1950s contributed to an increase in death rates. During each of these incidents, temperatures were lower than normal and the humidity was higher than normal—conditions ideal for upper respiratory infections among the elderly and the very young. The increase in death rates among these two groups following the "killer fogs" was entirely consistent with rates seen during episodes of similar temperature and humidity without industrial pollutants.
It is especially interesting to note a recent report on the autopsy findings on the frozen cadaver of an Eskimo woman who died some 16,000 years ago in Alaska. The cause of death was black lung disease, presumably the result of inhaling smoke from seal-oil lamps for a lifetime. No one today in the industrialized countries dies from air pollution created in their own homes by everyday living conditions.
Perhaps the worst environmental damage on earth today results, not from large-scale mining operations or from rubbish or sewage, but from deforestation in the Third World for cooking and heating purposes. The capital of Upper Volta, Ouagadougou, is a city of some 80,000 people. Not one bush or tree remains standing for a radius of 40 kilometers around the city; all the standing wood has been cut down for firewood, and there has been no effective reforestation program to compensate for the annual firewood consumption of approximately one ton per person just for cooking. The Himalayan foothills in Nepal, Pakistan, and parts of northern India are similarly being stripped, increasing erosion and aggravating flooding downstream, with disastrous effects on the best agricultural lands of India, Pakistan, and Bangladesh.
The burning of dried cow dung in India has accelerated since the OPEC oil price increases, reaching some 80 million tons per year in 1975. If this cow dung had been used for fertilizer instead of fuel, it would have supplied about one-third of India's national fertilizer needs. Greater affluence and the use of industrialized energy sources (coal, oil, hydroelectricity, geothermal wells, and nuclear reactors) avoids this kind of massive environmental damage. It is true, of course, that careful management of forests or village woodlots would provide a viable alternative in the Third World, but industrialization has nonetheless provided a way out.
Chemical spills and dumping into rivers, lakes, and the oceans are a potentially very severe environmental problem for the industrialized nations of the world. But just as in the Third World, the most effective and rapid solutions may well be political and legal rather than technological. The current approach in the United States is to provide for fines and penalties for violations of emission or release standards. The fines, however, are usually minuscule. A far more direct approach is to make each factory clean up its act by internalizing the cost of polluting the rivers: this can be done very easily by requiring each factory using river water to install its intake downstream from its waste pipes, using the same water supply for drinking water as well as industrial processes.
It should by now be clear that growing affluence and industrialization need not mean despoliation of the environment. In pragmatic terms, more affluent societies can afford greater aesthetic sensibilities with respect to the environment, and the historical trend has been just that.
THE MALTHUSIAN SPECTER As affluence has increased in the Western nations, death rates have declined, followed some time later by declining birth rates. The hope has been that the same would happen in the Third World as economic development brings greater literacy, more diversification of social and economic roles for individuals, and greater economic security in old age.
The common pattern in most impoverished countries is for a couple to have many children in order to ensure that, in the face of high infant and childhood mortality, at least one or two of their children will survive to adulthood to support the parents in their old age. Under these circumstances, the birth rate is, not surprisingly, high. If the infant mortality rate declines very gradually, public perception of that fact is long delayed, so the birth rate continues at the old levels much longer than is really necessary. But if the infant mortality rate changes abruptly and visibly, birth rates can and do change very rapidly as well.
In Sri Lanka (formerly Ceylon), the government instituted a program in the early 1970s in which every person (man, woman, and child) was guaranteed one kilo of rice free per week, with a second kilo available at a price substantially below the market price. The result was dramatic. Within two years, the birth rate had fallen by half, with many of the poor citing the improved health of their children as the reason for choosing to reduce the number of children conceived.
Feeding the poor does not simply breed more of them, as has been argued as far back as Malthus. Adequate food for the world's population, whatever it may be, is essential to any desirable future, and no intrinsic obstacles or limitations are in sight.
BEHIND BIRTH RATES With the advent of modern contraceptive technologies, many advocates of zero population growth have urged increased access and universal education in their use as essential programs for survival. Often they have advocated the use of compulsion or, at least, of severe economic penalties or incentives as tools for population policy.
Generally, these approaches have ignored the importance of old-age security as a motivation for bearing many children, often assuming that the high birth rates resulted from ignorance or inability to exercise personal choice and control over reproduction. But the actual facts show that the opposite is true, even in the absence of newer technological means of fertility control.
In the United States, multiple studies of human fertility have shown that, regardless of the technique used by a couple to prevent conception of an additional child, conception would eventually result if either parent had any ambivalent feelings about the desirability of an additional child. But if both parents were thoroughly determined not to have additional children, almost any method would work satisfactorily. And the experience of Sri Lanka shows how rapidly people can alter fertility patterns, even in the absence of technological birth control methods.
MINERAL RESOURCEFULNESS The ultimate threat of the Doomsday Mystique has been the imminent depletion of the minerals upon which industrial, technological society is based. The Limits to Growth was based on a computer model that incorporated this ultra-Malthusian assumption about raw materials. This assumption led, in that study, to the collapse of the socioeconomic system early in the next century—depletion of ores mined today would force us to mine lower-grade ores at much greater cost; eventually, the economy's supply of capital would be completely tied up in mining, leaving no capital for manufacturing and services. Soaring death rates and starvation would result, in this scenario, and nothing can avert this disaster save draconian measures to impose zero economic growth, zero population growth, and total recycling.
But the question of mineral resources was very poorly understood by the authors of The Limits to Growth. They underestimated the ability of technology to make substitutions when the price is right, to develop new, less expensive techniques for mining and refining ores when economic justification arises. Simultaneously, they overestimated the economic impact that an increase in the price of iron or lead or copper would have on the total economy.
In the United States, for example, the total value of new mineral production in 1968 was about 4 percent of the gross national product. If the price of all minerals, across the board, were to double or quadruple, the effect on the economy as a whole would not be disastrous, provided only that it happened sufficiently gradually. Over the period 1870 to 1957, at any rate, the average cost of minerals in the United States (when corrected for inflation) actually decreased, despite the fact that the grades of ores mined continued to decrease through most of the period for most minerals.
The table on page 22 gives us some clues about mineral consumption patterns. These figures, for the United States in 1975, reflect only virgin ore use; no allowance is made here for significant recycling of steel and aluminum, especially in industry. Some important points should be noted. First, metals are a tiny fraction of total minerals production and are overwhelmingly dominated by iron, the principal ingredient of steel. Second, fossil fuels amount to nearly half the total tonnage of minerals produced. (These figures are based on consumption in the United States, not extraction, so that our large petroleum imports are included here.)
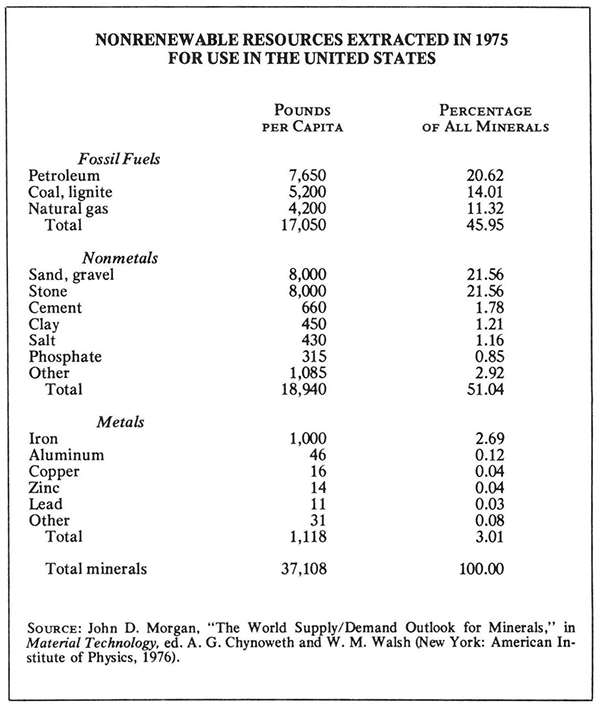
Additional data indicate that all of the materials that account for more than a tiny fraction of our total minerals consumption are available in sufficient supply, in forms accessible to present or near-term extractive technologies, to last for millions of years, with only two exceptions: fossil fuels and phosphate rock (used principally as a fertilizer in agriculture). Minerals that do appear to be intrinsically scarce (such as copper, zinc, lead, mercury, silver, colombium, and tungsten) must be examined case by case to see what effects we would suffer if they were to become unavailable. In each case, technological substitutions of abundant materials are available or could be developed within the present or near-term state of the art. In recent years, for sound environmental reasons, many of the former uses of lead have been eliminated, and substitutes have been found for each of these uses without perceptible increases in costs. A number of these metals are used in steel making to produce high-strength or chemically resistant alloys. For most of these applications, titanium would perform equally well, sometimes better, and is available in quantities comparable to aluminum.
What about the increased energy requirements for mining lower-grade ores? When the world's supply of bauxite (from which aluminum is presently extracted) is exhausted, we can turn to our choice of more than a dozen alternative minerals for which the technology for extraction is already known. In no case would the energy per finished ton of aluminum exceed the present energy expended by more than a factor of two. For iron, which dominates all metal production, the increased energy costs are even more moderate. Presently, we obtain most of our iron from taconite, which contains about 15 percent iron by weight. Known taconite reserves are sufficient for perhaps 1,500 years at present rates, but when they have been exhausted we can use lateritic iron ores, which contain about 5 percent iron and appear to be sufficient for several million years. The energy per ton of iron from laterites would be only 17 percent higher. Even without scrupulously recycling every gram of minerals used by society, we can confidently state that we are not doomed in the near future by minerals depletion.
But let's return to the other two categories of materials in short supply, phosphate rock and fossil fuels. Our agricultural system concentrates phosphorus in animal bones. Closed-environment agriculture can be expected in the not-too-distant future to significantly reduce consumption of all agricultural fertilizers, including phosphates. Should this not suffice, recycling of bones (especially by meatpacking houses) would make a major contribution. Ultimately, phosphorus can be extracted from ocean water should these measures prove insufficient. Distillation techniques would be very expensive (at least in terms of energy consumption) but would not bankrupt the economy, since phosphate is such a minute portion of the costs of agriculture. It is more likely, however, that within the next thousand years some selective ion-exchange process or osmotic membrane will be developed, allowing us to extract phosphorus efficiently and cheaply from ocean water.
BURYING THE FOSSILS Everyone agrees that we have to get off the fossil-fuels kick of the past two centuries—not so much because we are running out, but because of the accumulation of carbon dioxide in the atmosphere. The dynamics of the process are poorly understood at present, but we have some reason to believe that continued dependence on fossil fuels could, in two more centuries, raise the mean temperature of the biosphere by six to eight degrees centigrade because of increased opacity of the atmosphere to infrared radiation (the so-called greenhouse effect).
An additional important consideration is that in the United States today each ton of coal extracted from the earth produces some eight tons of mine wastes, which are a source of considerable quantities of biotoxic pollutants leached out by rain into nearby waterways. These pollutants include sulfuric acid, which is also a byproduct of copper mining. But the wastes from coal mining presently exceed those from the mining of all metals by a factor of 20. Once we make the transition from fossil fuels to long-term energy sources, pollution will be significantly decreased, even if population grows a few times larger and if we are reduced to lateritic ores for iron.
The present estimates for the length of time our fossil fuels would last do not include low-grade oil shales, as these are very expensive to extract (within presently foreseen technologies) in comparison with current sources of energy. In the long run, however, these oil shales may prove to be important as the basis for a continuing, more sophisticated petrochemicals and plastics industry. For such purposes, it is irrelevant whether the energy expended to obtain a kilogram of oil is less than or greater than the energy content of the extracted oil. Long before we must turn to such sources of hydrocarbons, however, it seems likely that genetic engineering or selective breeding of certain plants may provide us with agricultural supplies of complex hydrocarbons.
Thus, from a materials viewpoint, it seems that the earth itself can support perhaps 10 to 20 billion people—at a standard of living comparable to that in the United States today—far into the future, perhaps several million years. But a necessary development is a long-term source of abundant, environmentally clean, and inexpensive energy.
GETTING UP THE ENERGY The space enthusiast, of course, has a ready answer for the long-term energy needs of terrestrial civilization—the solar power satellite, which collects solar energy in space and converts it into electricity that is used to power a microwave-transmitting antenna array, to form a microwave beam focused on a receiving antenna farm on the ground for conversion back into electricity. The electricity can be used directly, with distribution through ordinary electrical transmission lines, or it can be used to synthesize gaseous and liquid fuels or to synthesize ammonia for fertilizer. Some of the fuel options that have been discussed include hydrogen (by electrolysis from water) and methanol (by high-temperature reaction of water and carbon dioxide, which can be extracted from air). Presently, it is unlikely that these would be highly significant in the early stages of space industrialization in the context of economic conditions in North America, but they could be highly relevant in the Third World, where the average cost per unit of energy in the form of liquid fuels is three to five times higher. In the long range, however, the "hydrogen economy" may prove to be most viable, since the transportation costs per unit of energy transported are considerably lower for pipelines carrying hydrogen than for electrical transmission lines.
But other options must also be considered in the long range. Fusion power has many attractive features. The local thermal load on the environment would be considerably higher than for solar power satellites but not high enough to be globally significant. Even if global energy consumption per capital rose to US levels for a population of 10 to 20 billion, the total thermal energy released would still be a tiny fraction of one percent of the solar insolation. Local effects, however, could be large.
A great deal of speculation has accompanied discussions of ground-based solar energy schemes. Space heating and cooling and water heating certainly are viable candidates for extensive use of solar energy, but so far solar energy to produce electrical power has been stymied by the need for large storage systems to carry through the night or through periods of heavy overcast.
One solar energy technology that circumvents this difficulty—photoelectrolysis—has received little attention but is amenable to sudden breakthrough. Photoelectrolysis is a process combining the energy of two photons to split a molecule of water into oxygen and hydrogen, which are released at physically separate plates. Because it can utilize low-energy photons, the process has theoretical efficiencies of 40 to 45 percent in converting solar energy into chemical energy in the form of hydrogen gas. The principal technical obstacle to be overcome is a problem with the plates at which the gases are liberated. The cathode, where single oxygen atoms are released, is subject to severe corrosion. The search for suitable plate materials or coatings for the plates is very similar to Thomas Edison's search for a material from which to make filaments for light bulbs. With the right materials, costs for hydrogen produced by photoelectrolysis could be comparable to, or cheaper than, costs per unit of energy for petroleum. Electrical power plants burning hydrogen and either air or oxygen would be environmentally very clean and very simple, requiring only half the capital costs per kilowatt of installed capacity of nuclear power plants. This would create a more difficult competitor for solar power satellites than is provided by nuclear power.
One point should be made here about solar energy systems of any type based on the ground. It is often claimed that such energy systems have no adverse environmental impact at all, but this is simply not true. It is true that solar energy farms in the American desert would not create a thermal source as intense as a nuclear (fission or fusion) power plant. But it is essential for any solar energy scheme to maximize the fraction of incident solar photons which are absorbed. Such collectors would thus increase the thermal burden of the biosphere compared to an equal area of barren desert sand, which reflects most of the sunlight falling on it. Reflected photons do not produce heat; absorbed photons do.
WHY MOVE INTO SPACE? The Doomsday Mystique provided us with a tidy rationale for moving into space quickly and massively, but the facts do not justify that Mystique. Should we, then, go into space in a big way?
Basically, only a handful of long-term goals can be convincingly demonstrated as valid motivations for this undertaking. First, such a move would provide "global insurance" for human civilization and for terrestrial life (including a wide variety of life forms besides Homo sapiens)—insurance against total extinction by a global catastrophe of either human or natural origin. Second, space industrialization can counter the adverse environmental impact of terrestrial mining and manufacturing, even beyond the improvements we will obtain once we cease mining of fossil hydrocarbons for energy. Third, the move into space will provide satisfaction for the very real human need to explore. The Roman poet Ovid, some 2,000 years ago, in describing the gift of human consciousness, wrote,
God elevated the forehead of Man,
And ordered him to contemplate the stars.
In realistic terms, none of these motivations provides sufficient justification for an investment totaling several tens of billions of dollars. Nations, as well as individuals, can become "insurance poor," and global insurance, as a justification for space programs, would fail to pass this objection. But short-term motivations must also be considered, and these, it would seem, will suffice to establish the kind of beachhead in space necessary and sufficient to eventually meet all of these long-term goals. These justifications are primarily economic, with some political considerations of secondary importance.
First, space industrialization can make a profit at an early date in the field of information; communications, earth observations and monitoring, navigation and geolocation, and minerals prospecting. Communications will offer the greatest opportunities for commercialization in the 1980s, with rapid return on investment. Political considerations (such as US foreign aid and economic-development assistance programs for Third World nations) are likely to be significant factors in minerals-prospecting applications.
Second, the prospects for making new, improved, or cheaper products in the new environment—made accessible for materials processing by reduced costs for transportation to orbit—are very good, but difficult to pinpoint at this time. In the 1980s, however, Space Shuttle/Space-lab experiments should be able to identify one or more products that can be made in zero gravity at a profit. Production of such goods would gradually (over five to ten years) lead to the establishment of fairly extensive habitation facilities in low-earth orbit, should these not already exist because of the need to service and repair large communications satellites.
Third, political decisions might be made by the United States and the Soviet Union to deliberately transfer some of their military hardware spending to space programs to provide a rapid decrease in military escalation without severe domestic economic dislocation. This seems somewhat unlikely but is a distinct possibility.
Any of these programs would lead to the establishment of facilities in space and of advanced launch vehicles. Within the next two or three decades, the costs of going into space would be low enough to reduce the front-end costs of nonterrestrial mining to a level attractive to private investors. Asteroidal mining of nickel for importation to earth could thus become a reality by the turn of the century, even if solar power satellites prove not to be economically or environmentally sound. Space tourism will be an inevitable spinoff of the reduced costs of transportation through space. Without solar power-satellites it is likely that the number of people living and working in space would not grow as rapidly. New commercial ventures and opportunities, however, can be expected to proliferate rapidly, once life in space becomes routine for even a few hundred people. The economy of North America diversified and proliferated independently of Europe once the Industrial Revolution arrived on its shores. The same is likely to happen with industries in space.
Why space industrialization? Because we want to go for the pure joy and fun of it, and we can pay our way while we do it. Whether or not we go, the long-term prospects for terrestrial civilization seem to be very good. Political factors (such as the possibility of a nuclear holocaust) remain, as they have always been, the wild card in the deck. As a space enthusiast, however, I feel I can face the future with confidence: we're on our way.
J. Peter Vajk has a Ph.D. in theoretical physics from Princeton. For eight years at the Lawrence Livermore Laboratory and now at Science Applications, he has been involved in studying space colonization and industrialization. He is the author of the recently published Doomsday Has Been Cancelled. This article is adapted from one published in Advances in the Astronautical Sciences, vol. 36, © 1978 by the American Astronautical Society.
This article originally appeared in print under the headline "No More Doomsday."
Hide Comments (0)
Editor's Note: As of February 29, 2024, commenting privileges on reason.com posts are limited to Reason Plus subscribers. Past commenters are grandfathered in for a temporary period. Subscribe here to preserve your ability to comment. Your Reason Plus subscription also gives you an ad-free version of reason.com, along with full access to the digital edition and archives of Reason magazine. We request that comments be civil and on-topic. We do not moderate or assume any responsibility for comments, which are owned by the readers who post them. Comments do not represent the views of reason.com or Reason Foundation. We reserve the right to delete any comment and ban commenters for any reason at any time. Comments may only be edited within 5 minutes of posting. Report abuses.
Please
to post commentsMute this user?
Ban this user?
Un-ban this user?
Nuke this user?
Un-nuke this user?
Flag this comment?
Un-flag this comment?